Energy Harvesting
Staff Members of the Project
- Prof. Dr.-Ing. Robert Schober
- Derrick Wing Kwan Ng, Ph.D.
- Diomidis Michalopoulos, Ph.D.
- Rania Morsi, M.Sc
1. Green Communication
Recently, green communication has received considerable attention because of environmental concerns. In particular, the cellular networks consume world-wide approximately 60 billion kWh per year. 80% of the electricity in cellular networks is consumed by the base stations (BSs) which produce over a hundred million tons of carbon dioxide per year. These figures are projected to double by the year 2020 if no further actions are taken. Hence, especially in the case when BSs are not connected to the power grid, energy harvesting is very appealing since BSs can harvest energy from natural renewable energy sources such as solar, wind, and geothermal heat, thereby reducing substantially the operating costs of the service providers.
Although the amount of renewable energy is potentially unlimited, the intermittent nature of energy generated by a natural energy source results in a highly random energy availability at the BS. For example, solar energy and wind energy are varying significantly over time due to weather and climate conditions. In other words, a BS powered solely by an energy harvester may not be able to maintain a stable operation and to guarantee a certain quality of service. Therefore, a hybrid energy harvesting system design, which uses different energy sources in a complementary manner, is preferable in practice for providing uninterrupted service.
Due to the time varying availability of the energy generated from renewable energy sources, the introduction of energy harvesting capabilities for BSs poses many new challenges for resource allocation algorithm design.

Literature:
[1] I. Ahmed, A. Ikhlef, D. W. K. Ng, and R. Schober, „Power Allocation for a Hybrid Energy Harvesting Relay System with Imperfect Channel and Energy State Information“, in Proc. IEEE Wireless Communications and Networking Conference (WCNC), Istanbul, Turkey, pp. 990-995, Apr. 2014.
[2] L. Xiang, D. W. K. Ng, W. Lee, and R. Schober, „Optimal Storage-Aided Wind Generation Integration Considering Ramping Requirements“, in Proc. IEEE International Conference on Smart Grid Communications (SmartGridComm), Vancouver, Canada, pp. 648-653, Oct. 2013.
[3] I. Ahmed, A. Ikhlef, D. W. K. Ng, and R. Schober, „Optimal Power Allocation for a Hybrid Energy Harvesting Transmitter“, in Proc. IEEE International Conference on Communications (ICC) 2013, Budapest, Hungary, pp. 4185-4190, Jun. 2013.
[4] I. Ahmed, A. Ikhlef, D. W. K. Ng, and R. Schober, „Power Allocation for a Hybrid Energy Harvesting Transmitter“, IEEE Transactions on Wireless Communications, vol. 12, pp. 6255-6267, Dec. 2013.
2. Wireless Information and Power Transfer
Conventional natural energy resources, such as solar and wind energy, are weather- and location-dependent, which may be unsuitable for mobile communication devices deployed in modern communication networks. An energy harvesting technology that overcomes the above limitation, is wireless power transfer, where the nodes charge their batteries from electromagnetic radiation.
-
Block diagram of a typical power scavenging module powering a communication transceiver.
Radio frequency energy harvesting (RF EH) provides the possibility for simultaneous wireless information and power transfer (SWIPT), since RF signals carry both information and energy. The integration of RF EH into communication systems brings some fundamental changes to the design of wireless communication networks.
SWIPT technology has promising applications in areas that can benefit from ultra-low power sensing devices. Potential applications include:
- structure monitoring by embedding sensors in buildings, bridges, roads, etc.,
- building automation through smart sensors that monitor and control different building processes,
- healthcare monitoring using implantable bio-medical sensors.
Literature:
[5] I. Krikidis, S. Timotheou, S. Nikolaou, G. Zheng, D. W. K. Ng, and R. Schober, „Simultaneous Wireless Information and Power Transfer in Modern Communication Systems“, IEEE Communications Magazine, vol. 52, pp. 104-110, Nov. 2014.
2.1. SWIPT for OFDM Networks
Orthogonal frequency division multiple access (OFDMA) is a viable multiple access scheme for spectrally efficient communication systems due to its flexibility in resource allocation and ability to exploit multiuser diversity. On the other hand, the increasing interest in high data rate services such as video conferencing and online high definition video streaming has led to a high demand for energy. This trend has significant financial implications for service providers due to the rapidly increasing cost of energy.
Literature:
[6] D. W. K. Ng, E. S. Lo, and R. Schober, „Energy-Efficient Resource Allocation in Multiuser OFDM Systems with Wireless Information and Power Transfer“, in Proc. IEEE Wireless Communications and Networking Conference (WCNC) 2013, Shanghai, China, pp. 3823-3828, Apr. 2013.
[7] D. W. K. Ng, E. S. Lo, and R. Schober, „Energy-Efficient Power Allocation in OFDM Systems with Wireless Information and Power Transfer“, in Proc. IEEE International Conference on Communications (ICC) 2013, Budapest, Hungary, pp. 4125-4130, Jun. 2013.
[8] D. W. K. Ng, and R. Schober, „Spectral Efficient Power Allocation in OFDMA Systems with Wireless Information and Power Transfer“, invited paper, 21st European Signal Processing Conference, Marrakech, Morocco, Sep. 2013.
[9] D. W. K. Ng, E. S. Lo, and R. Schober, „Wireless Information and Power Transfer: Energy Efficiency Optimization in OFDMA Systems“, IEEE Transactions on Wireless Communications, vol. 12, pp. 6352-6370, Dec. 2013.
2.2. SWIPT for Multiple Antenna Systems
Because of severe path loss attenuation, the efficiency of SWIPT technology is in general unsatisfactory for long distance transmission unless advanced resource allocation and antenna technology can be combined.
MIMO can be exploited to bring two distinct benefits to SWIPT networks. On the one hand, due to the broadcast nature of wireless transmission, the use of additional antennas at the receiver can yield more harvested energy. On the other hand, the extra transmit antennas can be exploited for beamforming, which could significantly improve the efficiency of information and energy transfer.
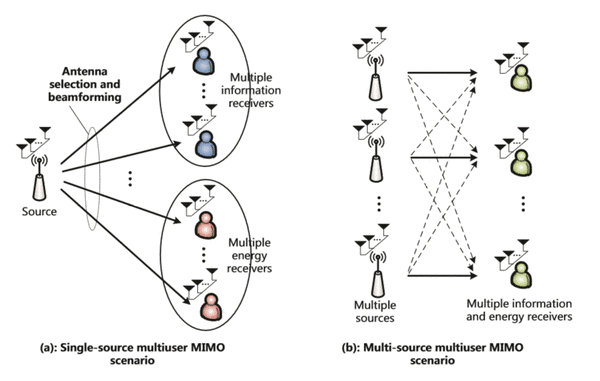
Literature:
[10] Z. Ding, C. Zhong, D. W. K. Ng, M. Peng, H. A. Surawerra, R. Schober, and H. Vincent Poor, „Application of Smart Antenna Technologies in Simultaneous Wireless Information and Power Transfer“, IEEE Communications Magazine, vol.53, no.4, pp.86-93, April 2015.
2.3. Physical Layer Security
Higher transmit power, which facilitates energy transfer from the transmitter to the receivers, leads to a larger susceptibility for information leakage due to the broadcast nature of wireless channels. Possible remedies include:
- Energy signal: Transmitting an energy signal along with the information signal can be exploited for expediting EH at the receivers. If the energy signal is a Gaussian pseudo-random sequence, it serves as interference to potential eavesdroppers, therefore it can be used to provide secure communication. If the sequence is known to all legitimate receivers, the energy signal can be cancellated at the legitimate receivers before information decoding.
- Jamming: Nodes in a network acting as jammers can be wirelessly charged by the RF signals sent by the legitimate users. The efficiency of this harvest-and-jam strategy depends on the network topology. In particular, harvest-and-jam nodes need to harvest a sufficient amount of energy. To overcome this problem, advanced multiple-antenna technologies are needed.

- Literature:
[11] D. W. K. Ng, E. S. Lo, and R. Schober, „Robust Beamforming for Secure Communication in Systems with Wireless Information and Power Transfer“, IEEE Transactions on Wireless Communications, vol. 13. pp. 4599-4615, Aug. 2014.
[12] D. W. K. Ng, E. S. Lo, and R. Schober, „Multi-Objective Resource Allocation for Secure Communication in Cognitive Radio Networks with Wireless Information and Power Transfer“, accepted, IEEE Trans. on Veh. Technol., May 2015.
[13] D. W. K. Ng, R. Schober, and H. Alnuweiri, „Secure Layered Transmission in Multicast Systems with Wireless Information and Power Transfer“, in Proc. IEEE International Conference on Communications (ICC), Sydney, Australia, pp. 5389-5395, Jun. 2014.
[14] S. Leng, D. W. K. Ng, and R. Schober, „Power Efficient and Secure Multiuser Communication Systems with Wireless Information and Power Transfer“, in Proc. IEEE International Conference on Communications (ICC), Sydney, Australia, pp. 800-806, Jun. 2014.
[15] D. W. K. Ng, and R. Schober, „Max-min Fair Wireless Energy Transfer for Secure Multiuser Communication Systems“, in Proc. IEEE Information Theory Workshop (ITW), Hobart, Tasmania, Australia, pp. 326-330, Nov. 2014.
[16] D. W. K. Ng, L. Xiang, and R. Schober, „Multi-Objective Beamforming for Secure Communication in Systems with Wireless Information and Power Transfer“, in Proc. IEEE International Symposium on Personal, Indoor and Mobile Radio Communications (PIMRC), special session on physical layer security, London, United Kingdom. pp. 7-12, Sep. 2013.
[17] D. W. K. Ng, and R. Schober, „Resource Allocation for Secure Communication in Systems with Wireless Information and Power Transfer“, in Proc. IEEE Globecom. 2013 – Workshop on Trusted Communications with Physical Layer Security, Atlanta, USA, pp. 1251-1257, Dec. 2013.
2.4. Green SWIPT
The performance of wireless power/energy transfer systems is severely limited by the distance between the transmitter(s) and the receiver(s) due to the high signal attenuation caused by path loss and shadowing. Distributed antennas are an important technique for mitigating interference, reducing the network power consumption, and extending service coverage. A promising architecture for distributed antenna networks is to split the functionalities of the base station:
- A central processor (CP) performs the power hungry and computationally intensive baseband signal processing.
- Low cost remote radio heads (RRHs) are responsible for all simple RF operations such as analog filtering and power amplification.
The RRHs are distributed across the network and connected to the CP via backhaul links. This system architecture is known as cloud computing network. The distributed antenna system architecture reduces the distance between the transmitters and receivers. Yet, transferring the information data of all users from the CP to all RRHs, as is required for full cooperation, may be infeasible when the capacity of the backhaul links is limited. Hence, resource allocation for distributed antenna networks with finite backhaul capacity is another important research challenge.


Literature:
[18] D. W. K. Ng, and R. Schober, „Secure and Green SWIPT in Distributed Antenna Networks with Limited Backhaul Capacity“, accepted for publication, IEEE Transactions on Wireless Communications, May 2015.http://arxiv.org/abs/1403.0054
[19] D. W. K. Ng, and R. Schober, „Resource Allocation for Coordinated Multipoint Networks with Wireless Information and Power Transfer“, in Proc. IEEE Globecom 2014, Austin, TX, USA, pp. 4281-4287, Dec. 2014.
[20] D. W. K. Ng, E. S. Lo, and R. Schober, „Energy-Efficient Resource Allocation in OFDMA Systems with Hybrid Energy Harvesting Base Station“, IEEE Transactions on Wireless Communications, vol. 12, pp. 3412-3427, Jul. 2013.
[21] I. Ahmed, A. Ikhlef, D. W. K. Ng, and R. Schober, „Optimal Resource Allocation for Energy Harvesting Two-Way Relay Systems with Channel Uncertainty“, in Proc. IEEE Global Conference on Signal and Information Processing, Austin, Texas, USA, pp. 345-348, Dec. 2013.
3. Performance Analysis and Scheduling
Further research challenges:
- Joint power control and user scheduling: Opportunistic power control can be used to exploit the channel fading for improved energy and information transfer efficiency.
- Energy and information scheduling: For passive receivers (such as small sensor nodes) “harvest-then-transmit” design can be used, when uplink data transmission is only possible after harvesting a sufficient amount of energy from the RF in the downlink. The system throughput can be optimized by varying the amounts of time allocated for energy harvesting and information transmission.
- Successive relaying, where two relays listen and transmit in succession: When implemented in a SWIPT system, the inter-relay interference can be exploited as a source of energy.
- Full-duplex transmission: Full-duplex relaying can double the spectral efficiency and loopback interference can be exploited as an additional source of energy with the help of advanced MIMO solutions.
- Design of new SWIPT relaying protocols which take into account circuit power consumption, power amplifier inefficiency, energy storage losses, energy consumed for relay network coordination, and the extra energy cost associated with acquisition of channel state information.
Literature:
[22] M. Chynonova, R. Morsi, D.W.K. Ng, and R. Schober, „Optimal Multiuser Scheduling Schemes for Simultaneous Wireless Information and Power Transfer“, invited paper, 23rd European Signal Processing Conference (EUSIPCO), Nice, France, 2015.
[23] R. Morsi, D. S. Michalopoulos, and R. Schober, „Performance Analysis of Wireless Powered Communication with Finite/Infinite Energy Storage“, accepted for presentation at IEEE International Conference on Communications (ICC), London, UK, 2015.
[24] D. S. Michalopoulos, H. A. Suraweera, and R. Schober, „Relay Selection for Simultaneous Information Transmission and Wireless Energy Transfer: A Tradeoff Perspective“, accepted for publication in IEEE Journal of Selected Areas in Communications (IEEE JSAC), Special Issue on Wireless Communications Powered by Energy Harvesting and Wireless Energy Transfer, 2015.
[25] D. S. Michalopoulos, H. A. Suraweera, and R. Schober, „Simultaneous Information Transmission and Wireless Energy Transfer via Selecting One out of Two Relays“, in Proc. 6th International Symposium on Communications, Control, and Signal Processing (ISCCSP), Athens, Greece, pp. 318-321, May 2014.
[26] D. S. Michalopoulos, H. A. Suraweera, and R. Schober, „The Impact of Relay Selection on the Tradeoff Between Information Transmission and Wireless Energy Transfer“, in Proc. IEEE Globecom 2014, Austin, TX, USA, pp. 4191-4196, Dec. 2014.
[27] R. Morsi, D.S. Michalopoulos, and R. Schober, „Multi-user Scheduling Schemes for Simultaneous Wireless Information and Power Transfer“, in Proc. IEEE International Conference on Communications (ICC), Sydney, Australia, pp. 4994-4999, Jun, 2014.
[28] R. Morsi, D. S. Michalopoulos, and R. Schober „On-Off Transmission Policy for Wireless Powered Communication with Energy Storage“, in Proc. Asilomar Conference on Signals, Systems, and Computers, CA, USA, Nov. 2014.